The making of the gut
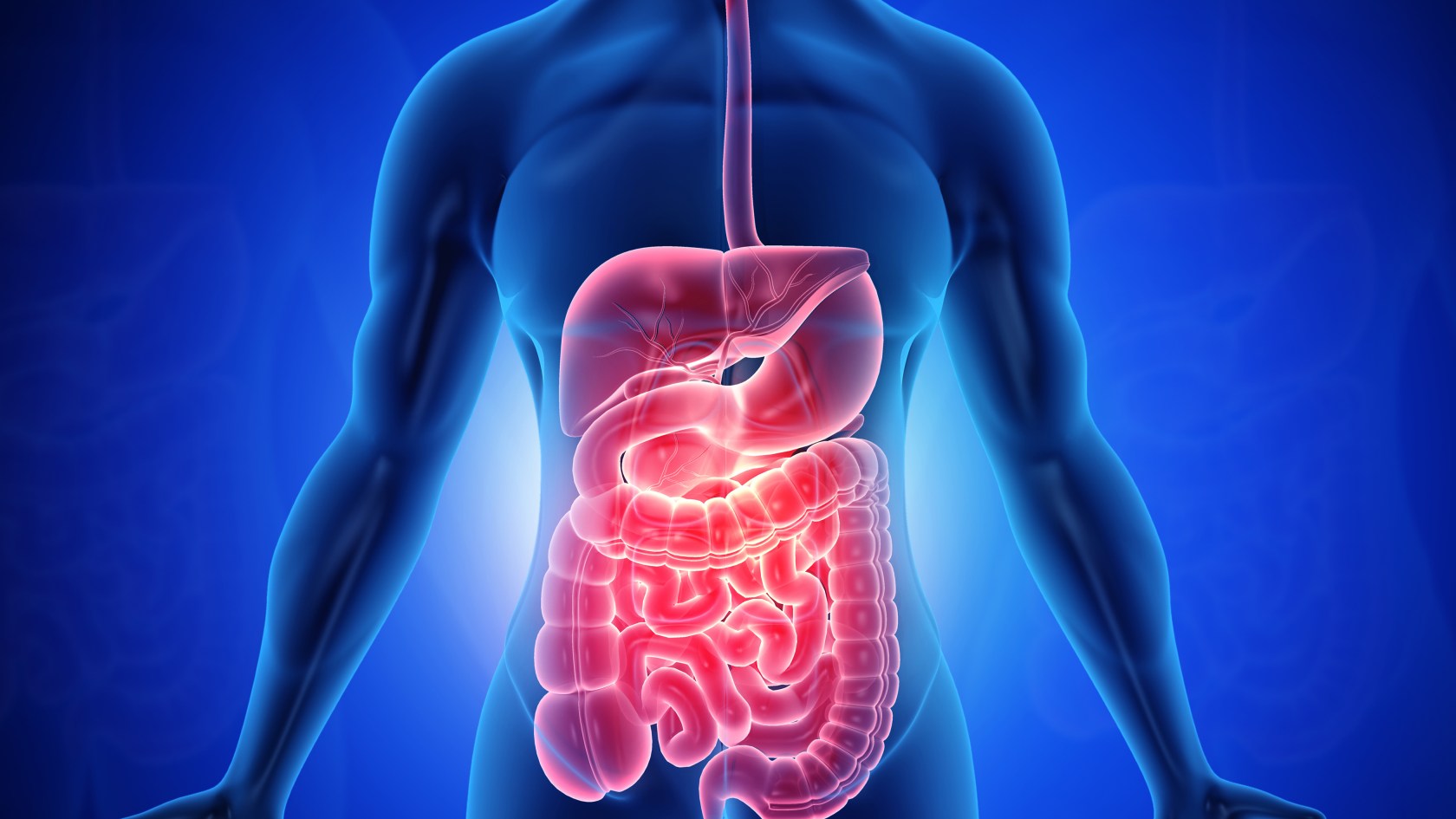
Studies connect genetics, physics in embryonic development
Genes are the control panel for an embryo morphing from a ball of cells into organs, muscles, and limbs, but there’s more involved than just genetics. There’s also physics — the shaping of tissues by flows and forces from cellular activity and growth.
Two recent studies in Developmental Cell and Proceedings of the National Academy of Sciences shed light on the gene-mediated geometries and forces within embryonic development that give rise to different sections and shapes of the gut, including the large and small intestines. The findings bridge a critical gap between genetic signals and the physical formation of the early gut.
The Developmental Cell paper, led by former Griffin Graduate School of Arts and Sciences student Hasreet Gill, shows how a set of developmental instructions called Hox genes dictate gut formation. For the study, Gill and colleagues traced the gut development of a chicken embryo as a model organism; Hox genes are also found in humans and all other vertebrates.
“I wanted to understand why different regions in the intestine, from the anterior, meaning esophagus, to the posterior, meaning large intestine, end up with different shapes,” said Gill, who co-authored both papers with her Ph.D. adviser Clifford Tabin, the George Jacob and Jacqueline Hazel Leder Professor of Genetics at Harvard Medical School. Gill was a student in the Department of Molecular and Cellular Biology’s Molecules, Cells, and Organisms program.
“I wanted to understand why different regions in the intestine, from the anterior, meaning esophagus, to the posterior, meaning large intestine, end up with different shapes.”
Hasreet Gill
The study connected experiment to computational theory through a collaboration with Sifan Yin, a former postdoctoral fellow in the John A. Paulson School of Engineering and Applied Sciences, and L. Mahadevan, professor in applied mathematics, physics, and biology in SEAS and FAS.
Gill’s study built on previous work looking at how Hox genes are involved in organ differentiation. The set of genes, highly conserved throughout animal evolutionary history, was the subject of the 1995 Nobel Prize when they were recognized for their role in segmenting a fruit fly’s body.
Gill and colleagues discovered that measurable mechanical properties of the tissues that make up the large and small intestines of a chick embryo are directly involved in how they arrive at their final shapes. For example, the tissues that form the villi located in the small intestine, she found, have different stiffness parameters than those that shape the inside walls of the large intestine, which form larger, flatter, more superficial folds.
To test the consequences of all these mechanical differences, the lab turned to its longstanding collaboration with Mahadevan’s lab, whose members, including Yin, carried out theoretical and computational analysis to define the impact of physical forces generated via differential growth on organ shape.
It had long been known that Hox genes are the instructions that lay the groundwork for how different organs, including the gut, are sectioned off and shaped. But the detailed “how” of this process had been a mystery.
To solve it, Gill and colleagues revisited a 1990s-era experiment from the Tabin lab that had investigated this question. In that experiment, they expressed a particular Hox gene in a small intestine and found it took on the characteristics of a large intestine.
Gill’s team repeated the experiment while running physical tests on the mechanical characteristics of the different parts of the gut, considering things like wall stiffness, growth rate, and tissue thickness. They found that the HoxD13 gene in particular regulates the mechanical properties and growth rates of the tissues that eventually lead to the large intestine’s final shape. Other, related Hox genes may define those same properties for the small intestine.
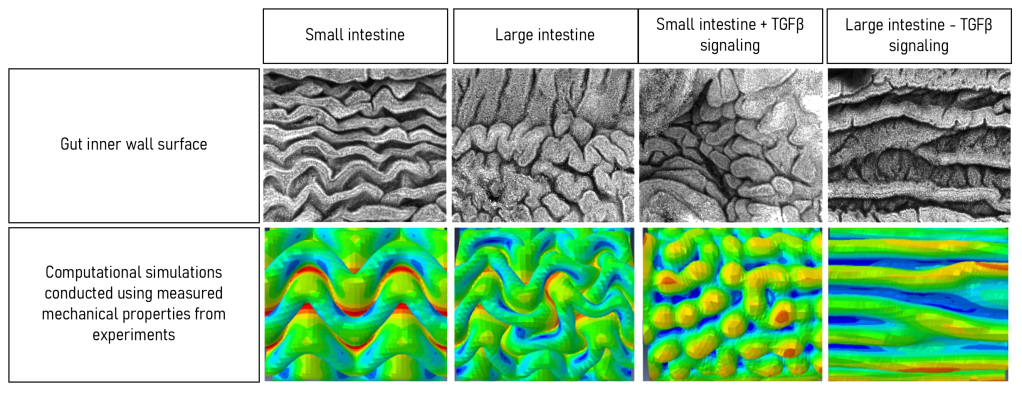
Crucially, they also illuminated the role of a downstream signaling pathway called TGF Beta, which is controlled by Hox genes. By tuning the amount of TGF beta signaling in their embryos, they could switch the shapes of the different gut regions. Seeing the importance of this pathway, long known to be involved in fibrotic conditions, was an important basic-science step toward fully understanding gut development in a vertebrate system.
These insights could lead to new knowledge of conditions for colon cancer and other fibrotic diseases of the gut, Gill said.
“One possibility is that the disease is co-opting a developmental program that can cause an excessive deposition of extracellular matrix, and this ends up being harmful to the patient,” she said. “Having this developmental context, especially related to Hox gene expression, might prove useful at least for understanding the broader context of why these diseases are happening in people.”
The complementary PNAS paper, co-led by Gill and Yin, showed how geometry, elastic properties, and growth rates control various mechanical patterns in different parts of the gut.
“We focused on how mechanical and geometric properties directly affect morphologies, especially more complicated, secondary buckling patterns, like period-doubling and multiscale creasing-wrinkling patterns,” said Yin, an expert in theoretical modeling and numerical simulations of active and growing soft tissues.
Added Mahadevan: “These studies allow us to begin probing aspects of the developmental plasticity of gut development, especially in an evolutionary context. Could it be that natural variations in the genetic signals lead to the variety of functional gut morphologies that are seen across species? And might these signals be themselves a function of environmental variables, such as the diet of an organism?”
Yin said the two papers provide a new paradigm for studying how genes affect the development of shape, or morphogenesis.
“Morphogenesis is driven by forces arising from cellular events, tissue dynamics, and interactions with the environment,” Yin said. “Our studies bridge the gap between molecular biology and mechanical processes.”