Probe the gut, protect the brain?
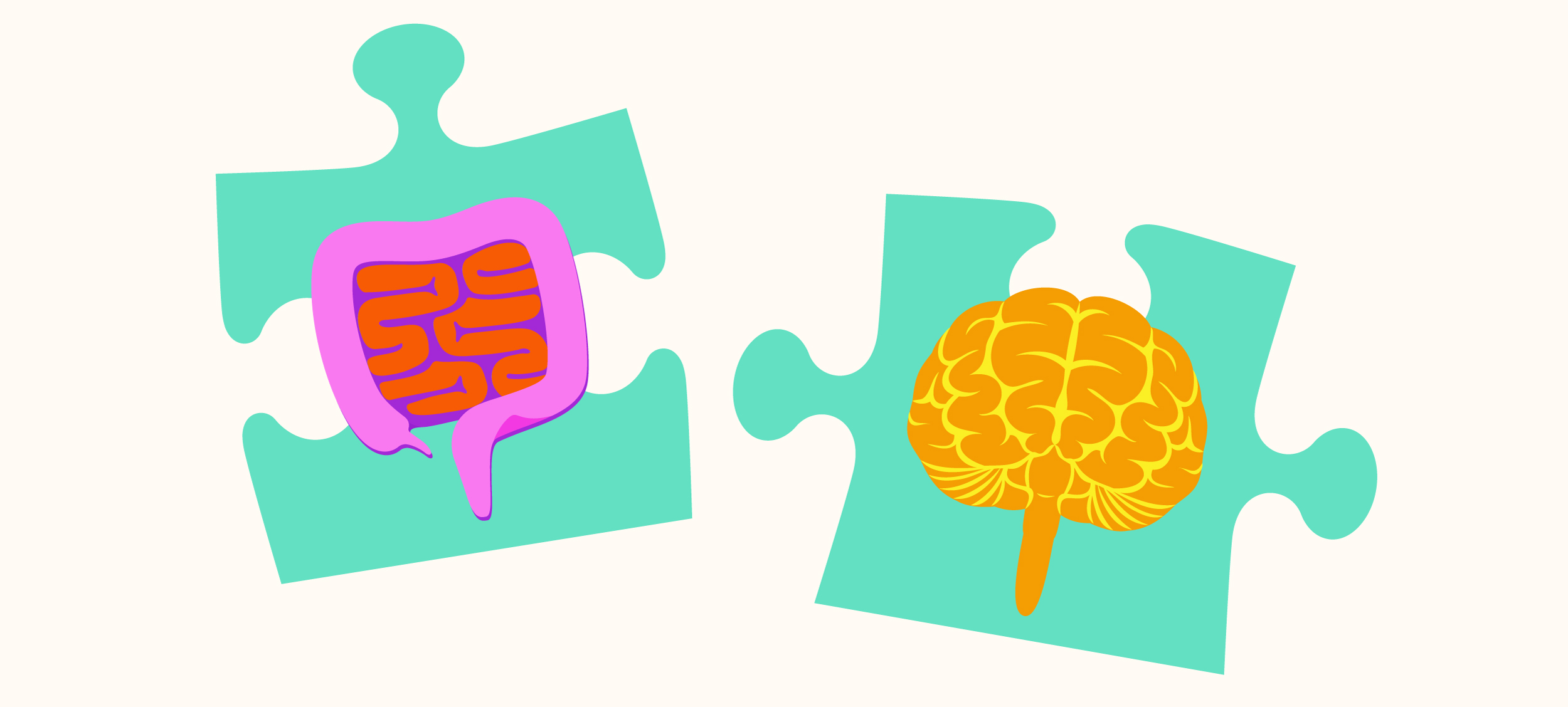
Illustrations by Judy Blomquist/Harvard Staff
In fight against Parkinson’s and other disorders, two-way connection may someday lead to a breakthrough
For Jo Keefe, the trembling hands and trouble walking were bad, but it was the nausea that was truly debilitating.
“For two or three years, I was having nausea for several hours every day,” said Keefe, a retired lawyer living in New Hampshire who suffers from Parkinson’s disease. “I’d wake up in the morning feeling sick and I couldn’t make any plans at all. Fortunately, I was retired, but I wasn’t planning on this for my retirement.”
Parkinson’s is a neurodegenerative disorder affecting cells that control movement. Patients and the doctors who treat them have long known that severe gastrointestinal issues — nausea, abdominal pain, diarrhea, constipation — are a feature of the condition, in some cases preceding neurological dysfunction by decades. But in recent years research around the disease has started to point to a connection that is more than incidental. The gut, experts say, may be where Parkinson’s starts.
Such a model, if supported by future research, would revolutionize our understanding of the nation’s second most common neurodegenerative disorder, opening a path for specialists to help patients like Keefe before neurological symptoms appear. It would also have the potential to inform treatment of other neurodegenerative disorders, including some of the most devastating in human health.
“What if you were able to get your screening colonoscopy and be told there’s a sign that you’ll progress to Parkinson’s unless we intervene now. And wouldn’t it be wonderful if we had a way to intervene now?”
Trisha Pasricha, specialist in neurogastroenterology and director of clinical research at Beth Israel’s Institute for Gut-Brain Research
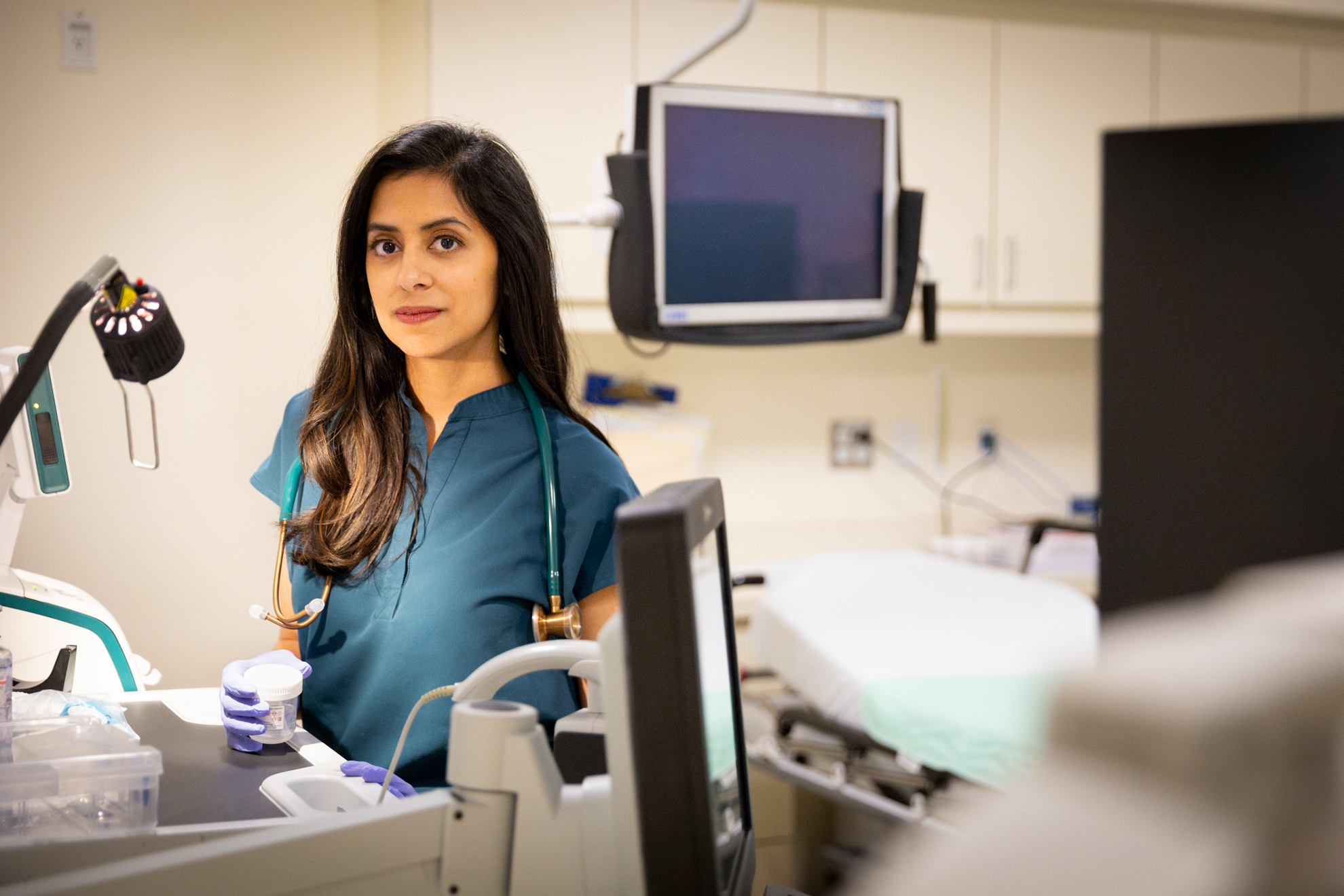
Trisha Pasricha.
Niles Singer/Harvard Staff Photographer
“Everyone’s goal is to find an early biomarker for Parkinson’s and our hope is that we can find one in the gut,” said Trisha Pasricha, a specialist in neurogastroenterology and director of clinical research at Beth Israel Deaconess Medical Center’s Institute for Gut-Brain Research. “What if you were able to get your screening colonoscopy and be told there’s a sign that you’ll progress to Parkinson’s unless we intervene now? And wouldn’t it be wonderful if we had a way to intervene now? There are many steps that need to happen, but that’s the goal.”
Central to Pasricha’s vision is the gut’s enteric nervous system, which contains as many neurons as the spinal cord and presides over digestive processes that function as the body’s intake department: proteins, carbohydrates, alcohol, drugs, fiber, agricultural pesticides, hormones given to livestock, chemicals used in food processing, bacteria, viruses, and on and on. The system processes signals about what we’ve consumed and how to respond: throw it back up or move it along; speed it up or slow it down.
Also key: a focus on the two-way nature of the gut-brain connection. Stress caused by the perception of potential danger can cause digestive ills, for example, while signals from the gut’s own nervous system, the enteric nervous system, can spur the brain into mobilizing the body via hunger, cravings, nausea, and pain.
“The enteric nervous system is this large network that runs throughout the gut,” Pasricha said. “It’s constantly signaling, influencing our mood, our wants, our needs. Some of the earliest animals had an enteric nervous system well before anyone developed a brain, well before anyone developed a central nervous system, because we all had to eat. It’s like the OG of our bodies.”
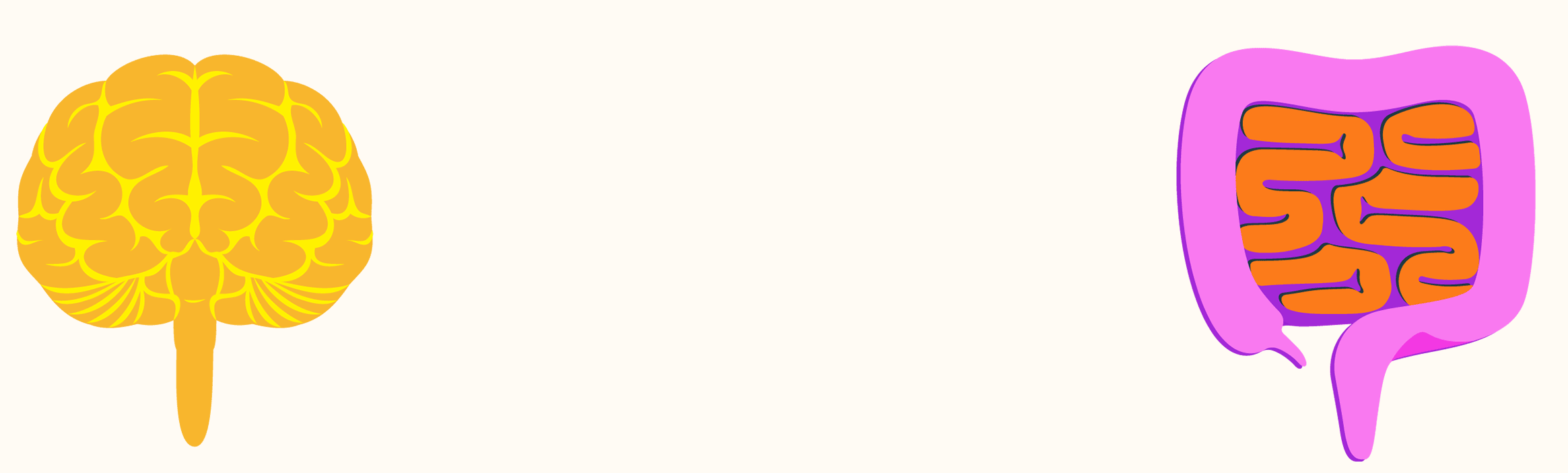
The gut-brain connection goes both ways. Stress caused by the perception of danger can cause digestive ills, for example, while signals from the gut’s enteric nervous system can spur the brain into mobilizing the body via hunger, nausea, and pain.
The gut is also home to the microbiome, a symbiotic community of thousands of species of bacteria and other microbes whose chemical byproducts promote health by protecting against pathogens and regulating immunity. Except, that is, when the balance fails, and symbiosis turns to “dysbiosis,” in which the chemicals released by our microbial companions interfere with healthful processes. Researchers have only scratched the surface of the microbiome’s complexity, but they’ve identified shifts in the gut microbial community — some bacteria populations rise and others fall — not only in Parkinson’s but in Alzheimer’s disease, multiple sclerosis, and amyotrophic lateral sclerosis.
“Parkinson’s disease is very well known and that galvanizes a lot of research,” Pasricha said. “What we often find in science is that when we understand mechanisms behind one disease, it teaches us lessons that we can apply to the other diseases too.”
Theories of the case
There is some variation in Parkinson’s disease, which affects nearly 1 million Americans, but the most common form of the condition, sporadic Parkinson’s, is believed to stem from a complex interaction of environmental and genetic factors.
The disease develops over decades and is caused by a misfolded protein — alpha-synuclein — accumulating in dopaminergic neurons, which play a key role in regulating movement, cognition, and emotion. This process leads to the disease’s characteristic tremors, followed by slowed movements, altered gait, and impaired balance. The impact on neck and facial muscles slurs speech. Patients can experience difficulty swallowing, leading, in later stages, to the need for a feeding tube. The degeneration can spread to other types of neurons and, in some cases, contribute to dementia.
In 2016, researchers examined samples of gut tissue taken from Parkinson’s patients before they developed symptoms. They found alpha-synuclein present in the gut as early as two decades before it appeared in the brain. Additional studies have offered clues to how the protein might travel to the brain, showing that peptic ulcer patients who underwent vagotomies — severance of the main nerve connecting the gut and the brain — experienced significantly lower incidence of Parkinson’s disease.
These findings have led some scientists to embrace the idea that alpha-synuclein appears first in the gut in some forms of Parkinson’s. There, the protein — or changes associated with it — may create disturbances in the enteric nervous system, causing severe constipation, gastroparesis, and other hallmark Parkinson’s gut symptoms. It then moves up the vagus nerve to the central nervous system, where it begins the assault that leads to neurodegeneration.
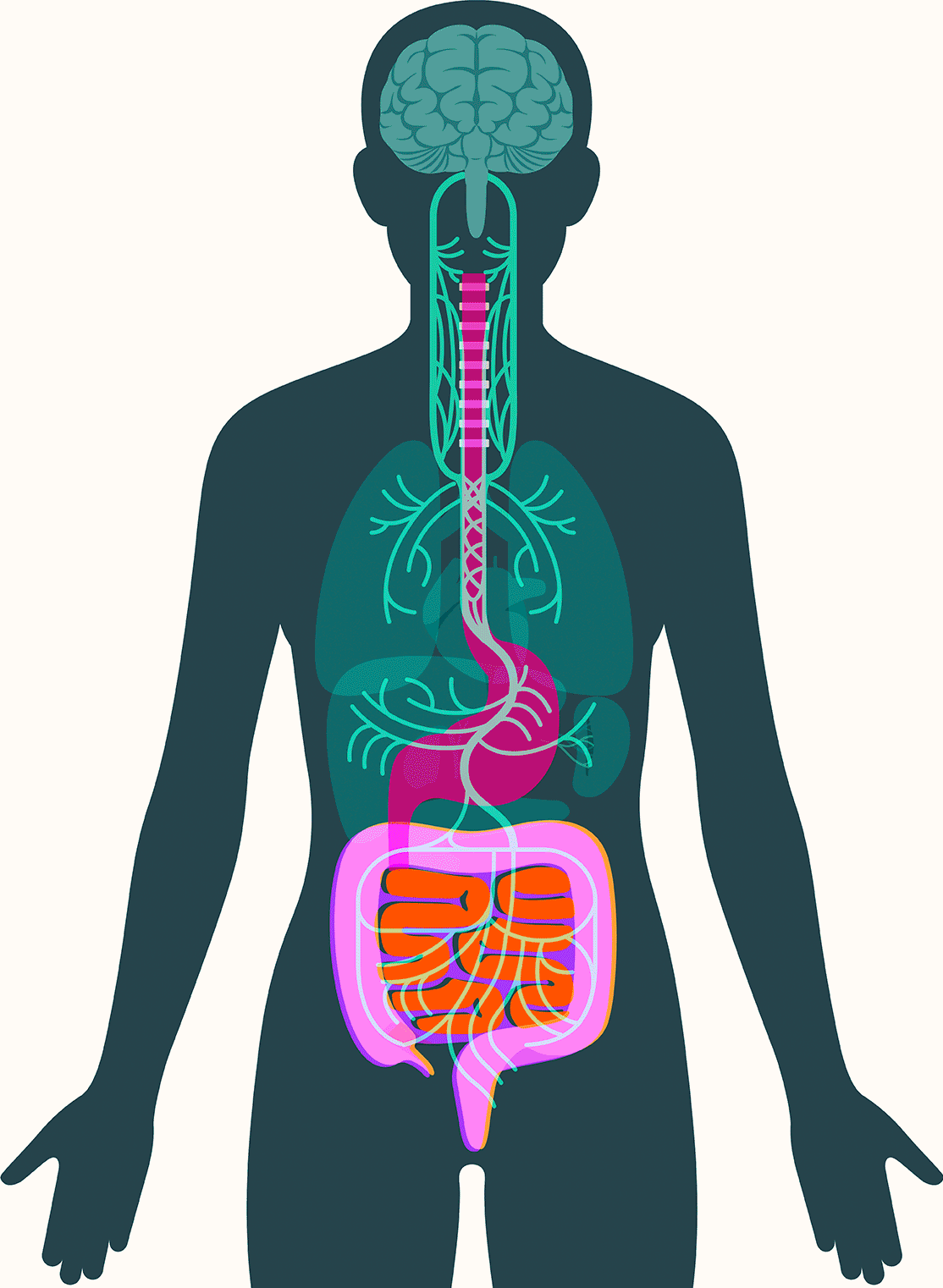
In September, Pasricha and colleagues added to that emerging picture, linking damage to the mucosa that lines the upper small intestine to Parkinson’s disease. The study, published in the Journal of the American Medical Association, found that among more than 9,000 patients with no signs of Parkinson’s when they were examined, those with mucosal damage experienced a dramatically increased risk — 76 percent — of later developing the disease.
Subhash Kulkarni, an assistant professor at Harvard Medical School and co-author on the paper, cautioned that while the results are intriguing, much work remains. Scientists still don’t know for sure what alpha-synuclein does in the gut, he noted, and the protein has also been found in the skin and salivary glands.
“These are initial forays,” Kulkarni said. “The relevance of these proteins in the gut to Parkinson’s is still not well understood.”
Beyond Parkinson’s
Laura Cox arrived at Brigham and Women’s in 2019 for postdoctoral studies on the microbiome, focusing on multiple sclerosis, a neurodegenerative condition in which the immune system attacks the myelin insulation that sheathes nerve cells. She worked in the lab of Howard Weiner, the Robert L. Kroc Professor of Neurology, who kept a plaque on his desk that read “Cure as many diseases as possible.” She took that admonition to heart.
“We said, ‘If we’re going to do the microbiome and MS, we’re going to work with our neighbors across the hall,’” said Cox, today an assistant professor of neurology at Harvard Medical School and the Brigham’s Ann Romney Center for Neurologic Diseases. “A really important thing that’s emerging is that there is clear evidence that the gut microbiome can influence neurologic disease.”
In addition to MS, Cox’s lab works on Parkinson’s, Alzheimer’s, and ALS, trying to decipher how gut microbes influence diseases that a few decades ago were thought to be confined to the brain and central nervous system. What she and other experts have found is that “dysbiosis” — shifts in the microbiome favoring one species of bacteria over another — occurs in each condition. And some of the same names keep popping up: Bacteroidetes, Akkermansia, Blautia, and Prevotella, among others.
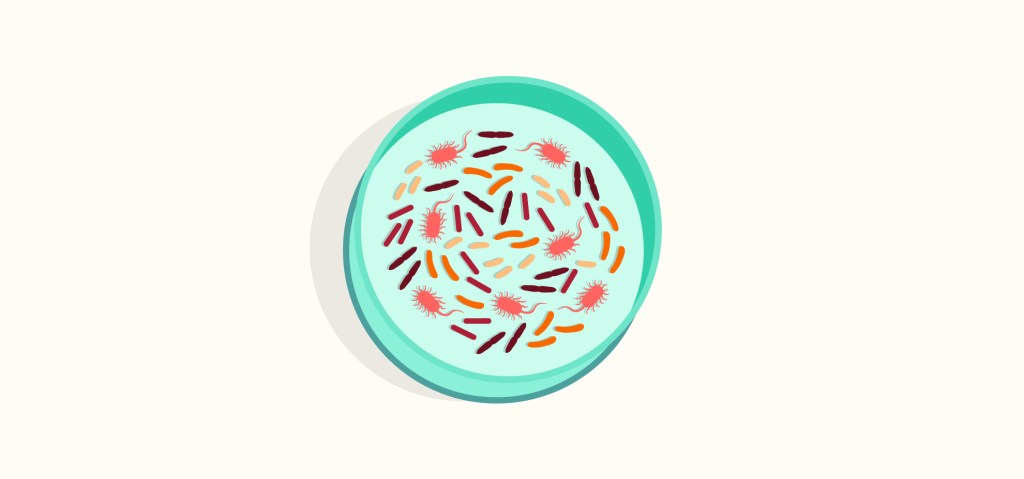
These bacteria ingest and secrete metabolites that protect or harm health as they live, reproduce, and die, and can trigger neurodegeneration in two major ways, according to Cox. They can interfere with immune function that otherwise might remove harmful proteins such as the amyloid beta that accumulates in Alzheimer’s disease. They can also boost inflammation, an important contributor to the neurological damage in Parkinson’s disease.
“What we found in Alzheimer’s was that Bacteroidetes drove immunosenescence and it blocked this important repair process in which microglia go into the brain and clear out plaques,” Cox said. “In Parkinson’s there’s really strong evidence that the gut microbiota contribute to disease by driving inflammation.”
There are three routes through which gut metabolites affect the brain, according to Francisco Quintana, a professor of neurology at the Brigham whose lab studies the gut-brain axis and neurodegeneration. As in Parkinson’s, they can travel via the nervous system and the vagus nerve. They can also move directly to the brain via the bloodstream, crossing the blood-brain barrier. Third, they can activate immune cells in the gut that travel to the brain and release signaling molecules called cytokines. Those molecules can also cross the blood-brain barrier and trigger the brain’s own immune cells into action.
“I don’t know if it is cause or consequence, but if we model that gut flora, there might be effects on central nervous system pathology — and I think that’s extremely exciting,” Quintana said. “The gut affecting our central nervous system health, our brain health, gives us a unique opportunity to track the brain.”
Forward thinking
In 2020, Aaron Burberry was a postdoctoral researcher in the lab of then-Harvard Professor Kevin Eggan, who had developed a strain of mice that replicated the rare but fatal neurological disease ALS.
Burberry and Eggan created a second population of mice for a lab at the Broad Institute of MIT and Harvard. These animals were genetically identical to the first set and exposed to similar environmental conditions — same food, same light-dark cycles — but they never developed the ALS-like immune response and nervous system inflammation of their predecessors. That divergence sparked a scramble to understand the difference between the two populations, with the evidence eventually pointing to the microbiome. Some microbes present in the guts of Harvard mice were absent in the Broad Institute mice, the researchers discovered.
Burberry and Eggan also found that manipulating the microbiome with antibiotics or fecal transplants from the Broad mice improved or prevented ALS symptoms in the Harvard mice. Burberry, now a professor at Case Western Reserve University, has built on those results, recently identifying a protein produced by immune cells in response to gut microbes that drives up an immune factor called Interleukin 17A, which triggers inflammation in the genetically engineered mice. The FDA has already approved a drug targeting IL-17A, for psoriasis and rheumatoid arthritis, that potentially could be repurposed for ALS. In addition, human trials testing fecal transplant in early ALS patients have begun in Europe.
Work toward gut-based therapeutics for other brain diseases is also moving forward. Rudy Tanzi, an Alzheimer’s specialist and the Joseph P. and Rose F. Kennedy Professor of Child Neurology and Mental Retardation at Harvard Medical School, is developing a “synbiotic” to boost microbiome health. The synbiotic combines probiotics — healthy bacteria — and prebiotics, high-fiber compounds that encourage their growth. Meanwhile, Quintana is using the tools of synthetic biology to engineer microbes — bacteria, yeast, and viruses — to deliver medication that tamps down inflammation before it becomes a problem.
“We might never be able to tell whether it is actually the microbiome exacerbating it or whether it is just reacting to a deeper perturbation in the body,” Quintana said. “But we can look: Is there something in the microbiome that I can use as a biomarker? Can we exploit the microbiome, or perturbations in the microbiome, to develop novel therapies?”